Discharge plasmas for generation of EUV light
EUV lithography systems
An essential difference between "conventional" systems for lithography and the future systems for EUV lithography, is that in the latter, lenses as optical elements are replaced by multilayer mirrors. This is necessary because suitable materials for making lenses (which are sufficiently transparent and have a high enough index of refraction) do not exist at wavelengths below about 150 nm. Because of the large number of reflective surfaces (12 or more) and the limited reflectivity of each surface (of no more than 70 percent), only a very small fraction of the EUV power emitted by the light source finally reaches the wafer surface. The result is that an EUV lithography system needs a light source that emits several hundred Watts of EUV in a narrow wavelength range of 2% around 13.5 nm.
Other required properties are a certain minimal lifetime, and sufficient cleanliness of the source: this means that debris (both microscopic and macroscopic) and fast particles produced by the source, should not limit the lifetime of the optics in the system too much.
Discharge sources of EUV radiation
At this moment no source exists yet that has been proven to meet all requirements. Several source concepts are being investigated that may potentially be developed further into a commercially applicable source. The various concepts can be roughly subdivided into two main groups: laser produced plasmas (LPPs) and discharge plasmas.
The research performed by TU/e in an EUV laboratory of ASML, focuses on two different types of discharge plasmas: a hollow-cathode triggered discharge of Philips EUV (see schematic) and a laser-ignited discharge in tin vapor, developed by the Institute of Spectroscopy in Troitsk, Russia.
Both discharge plasmas can be described as a very short-lasting discharge (with duration on the order of several hundred nanoseconds) over an electric potential of a few kilovolts, and with a gap between both electrodes of a few millimeters. Also the evolution of the plasma during the pulse has been observed to show strong similarities. In both cases, an electric current through the discharge of many kilo-amperes causes a Lorentz force that creates an implosion of the plasma in the radial direction, so that a plasma with a needlelike shape emerges on the axis of symmetry. For this reason, such discharges are also called "pinch plasmas".
The main differences between both types of EUV sources are the working element (xenon versus tin) and the way each discharge is ignited.
Experimental techniques
The experimental characterization of these plasmas, apart from the measurement of electric potentials and currents, and the absolute EUV energy emitted per pulse, is done in the first place by making time-resolved images of the plasma in the EUV, and by determining time-resolved EUV spectra. Because of the short pulse duration, a time resolution on the order of 5 ns is required for this.
By application of EUV imaging, it becomes possible to give a qualitative description of the evolution of the plasma. When such EUV "photos" are displayed one after another, the result is a short "movie" of the plasma pulse.
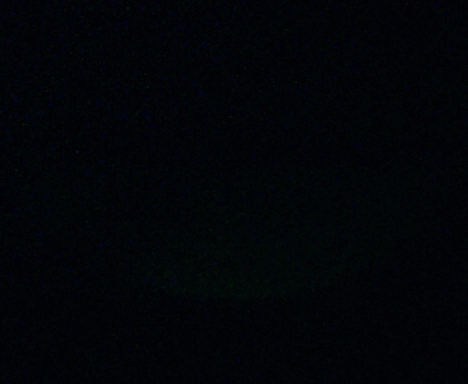
Analysis of the EUV spectrum leads to knowledge about the relative abundances of the different ionization stages of the chemical element in question, and, more indirectly, about the temperature and types of balances that govern the plasma.
More recently, the above-mentioned diagnostics have been supplemented within EINLIGHTRED with measurements of Stark broadening and Thomson scattering. These lead to more quantitative information about the plasma, mainly in the form of densities and temperatures of the present particles. To avoid the strong background radiation emitted by the plasma itself, in the latter technique, shorter laser pulses have to be applied than usual. To this end, a new setup has been built for measuring Thomson scattering, in which a camera that has a shutter time of just 200 ps can be syncrhonizaed to a laser pulse with a duration of 150 ps.
Plasma-wall interaction
To further reduce absorption losses in the lithography tool, its entire optical path is embedded in a vacuum system. Still, the background gas in the tool will get photo-ionized by the EUV radiation (see the illustration)
,
creating a low density weakly-ionized plasma (see, for example, the photograph below). The plasma can damage optical components in case high velocity plasma ions impact on the mirror surface.
A new branch of the research is therefore aimed at characterizing the EUV generated plasma, through Langmuir probe measurements and passive spectroscopy diagnostics, and at studying the effects of plasma ion bombardment on the mirrors with sensitive surface analysis techniques. To understand the dynamics of the bombardment process a kinetic plasma model has been developed. This Particle-In-Cell Monte Carlo model predicts the flux and energy of the plasma ions. Future plans focus on determining the threshold energy at which ions start to damage the mirrors.
Picture below: visualisation of a focused EUV beam through emission of light by background nitrogen gas.
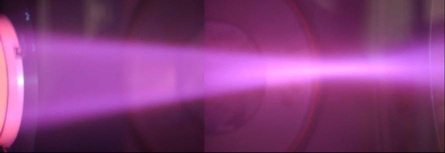
Further reading
Several publications are already available on the characterization of EUV sources. The articles listed below (all in pdf format) describe the different aspects.
The results of the experiments using "sub-nanosecond" Thomson scattering will be added here at a later time.
by Erik Kieft